The culmination : Creation of the WAC Corporal
by Benjamin Zibit
The following text is the chapter 13 of Benjamin Zibit's Ph.D. dissertation :
The Guggenheim Aeronautics Laboratory at Caltech and the Creation of the
Modern Rocket Motor: How the Dynamics of Rocket Theory Became Reality.
Graduate Faculty in History, City University of New York, February 1999.
The WAC Corporal, a sounding rocket just under 16. 2 feet high and a bit
over a foot in diameter, evokes an image of the nested Russian matyoshka, (wooden dolls) : smaller and smaller egg-like faces pop out as one opens one doll after another, each revealing a face smaller in size than the one before. Such is
the story of the WAC Corporal, a rocket with a tiny brother, appropriately named " Baby WAC ", and a much larger sibling, the Corporal ballistic missile. " Baby WAC " was only one-fifth the length of the WAC Corporal, or just over 3.2 feet
long. GALCIT designers built " Baby WAC " strictly to test the flight characteristics of a full-size WAC Corporal 1
. The Corporal, largest of the three rockets, when finally constructed, would measure 39 feet in length.
After returning from this fact-finding tour of Europe for Army Ordnance in December 1945, Frank Malina picked up a thread of secret rocket development that had been underway since August 1944. The ORDCIT project had not
merely developed and successfully test-fired for the benefit of Army Ordnance, entitled " Research Program for the Second Type of Long-Range Jet Propelled Missile (XF30L 20, 000) ", ORDCIT Memorandum No. 2 2. The memorandum outlined JPL's plan to engineer and build a liquid propellant rocket
with a 20, 000 lb. thrust potential, capable of reaching a target 30-40 miles down range. This missile, designated tentatively XF30L 20, 000, signifying both its power and its shell diameter (30 inches), was intended to be the fruit of
coordinated research by both GALCIT engineers and Aerojet. The drawing-board prototype, designed with an internal guidance system and equipped, either with a cluster of 3, 000 lb. -thrust Aerojet motors or one massive 20, 000 lb.-thrust
motor (not then developed), evolved into the Corporal rocket.The Corporal was the first operational ballistic missile in the US Army's arsenal. ORDCIT/ Aerojet teams designing the Corporal foresaw a test-firing date of March 1, 1945,
but this proved far too ambitious. The first Corporal E did not roll off the assembly line until May 1947, with its first test-firing almost immediately afterward at White Sands Proving Ground on May 22. The Corporal weighed 11, 700 lbs.
when loaded and could carry a "300-400 lb. payload up to 62.5 miles 3.
Its original specifications had called for a length more than two-and-one half times its actual dimension (100 feet), a range half its true potential, and a gross weight of 10, 000 lbs. 4 . Over various stages of its development XF30L 20, 000 slowly emerged from the
simultaneous effort of JPL engineers, who designed the missile's casing and calculated its trajectory and guidance system ; Aerojet's Liquid Propellant Rocket Section provided the missile's motor. The Aerojet/JPL link, now firmly cemented,
was gliding into a new realm of rocket construction. No longer would JATOs be the delivered product. A true missile would be born, the fruit of collaboration between the first American academic life : a joint Caltech/US military research
arm fashioned specifically to design rockets. " Phase 3 " of von Karman's planned program of rocket construction at Caltech and Aerojet was becoming a reality
5
. The gamble GALCIT's director had taken in 1936, risking his academic reputation on a small group of visionary engineering students and unpredictable rocket builders, had produced a dividend not even he could have predicted.
The WAC Corporal was Frank Malina's cherished creation. He and fellow engineer Homer Joe Stewart had hoped to generate Army Ordnance support for its construction with the completion of a January 1945 ORDCIT
memorandum, " Considerations of the Feasibility of Developing a 100, 000 Ft. Altitude Rocket (The " WAC Corporal ") " 6 . The bait worked, and according to Malina, Army Ordnance's Gervais Trichel then requested a JPL study of the proposal. Malina even called the WAC Corporal engineers " my WAC team "
7. Key among them were Stewart and Mark Mill, both of them GALCIT Ph.D.s,
both excellent rocket engineers. Malina admitted in his 1971 memoir that, " in 1936, I had placed on my office wall at CALTECH a chart showing the component parts required for a successful sounding rocket. The dream had now become a
reality, with an engineer in charge of each of those components." 8
However, reality was a bit more complex that Frank Malina's vision. Malina had shelved his dream while engineering and testing JATOs, and while building a company whose sole client was the military. It was actually the von
Karman memorandum of November 1943, along with Tsien and Malina's attached collaboration, " Review and Preliminary Analysis of Long-Range Rocket Projectiles ", which had spurred the WAC Corporal's development. The WAC Corporal, a smaller
version of the proposed 10, 000 lb. missile foreseen by von Karman, also had a military call-name. Although most knew the WAC as an acronym for the " Women's Army Corps ", an auxiliary of the army, to those in-the-know at JPL/GALCIT, WAC
also stood for " Without Attitude Control ", since the rocket had no internal guidance system 9. The problem of guidance was solved externally, by constructing long launch tower; with initial propulsion out of the tower using booster rockets whose purpose would be to catapult the WAC
Corporal to a speed of 400 fps., the minimum required for a nearly vertical trajectory. Although Malina usually referred to the WAC Corporal as a rocket whose payload was to be purely meteorological, even meant to be shot
off near populated areas as a weather forecasting and scientific research tool (close to the original expectations of Robert Millikan, who wanted a sounding rocket capable of carrying out cosmic ray research), the truth is that he sold the
idea for the rocket to the military by pointing out that the WAC Corporal " would represent a logical first step in the development of a guided anti-aircraft projectile . " 10 Malina had quite correctly criticized Goddard for failing to approach rocket research with
a true " team approach ". " The day of the isolated inventor of complex devices was over ", he wrote in 1971, musing about the birth of the WAC Corporal
11. In 1945, the only ready source of sufficient funds for the " team approach " was the military, and it was the military which seized upon the
weapon potential of the sounding rocket. Perhaps prophetically, in mid-1945, the US Army was also preparing to use the atom bomb on Japanese cities, in hopes of quickly ending the war in the Pacific. As for the WAC
Corporal and its future, Malina had little choice but to work with the military. The Jet Propulsion Laboratory, at least partially the brain child of his own efforts and research, had become a government-owned facility. His own university
Caltech, his home since the mid-1930s, was linked to the everyday management of JPL and the Institute exerted influence upon JPL's policy evolution. Malina's efforts, born of his love for pure science in 1936, had led him and his team to
collaboration with the armed forces. He disliked intensely their penchant for secrecy ; the implications of rocket research for future international political stability had begun to dawn on him. However, the only way for
Malina to build the sounding rocket without military support would have been to resign from Caltech and begin work with an amateur rocket society, such as the ARS. Other technical institutes, like MIT, were themselves deeply involved in
military-related research. If one wanted to build state-of-the-art rockets in 1945, apparently the only road to follow was the military road. The engineering of the WAC Corporal was actually quite a remarkable
achievement. Only a collaborative effort by several scientists and technicians could have produced it. The performance characteristics of both the Private A and the Corporal missiles had been worked out, at least in theory, by Malina and
Tsien in their November 1943 report on Long-Range Rockets. The two engineers were able to compute range and thrust considerations for the Private A, creating a set of velocity equations that would hold for a variety of flight angles
12. These equations were extended and modified to allow for
measurement of thrust (specific impulse) for the Corporal. Difficulties arose because there were no specific calculations available for a rocket intermediate between the Private A and the Corporal. This was odd, as the
closest specifications described in the 1943 report were for a liquid propellant rocket with an initial weight of 2000 lbs. and a 4000 lb.-thrust capacity (LRRP-II) 13. The WAC Corporal fell far short of this, with a loaded weight of 665 lbs. and a thrust of 1, 500
lbs. It seems that, prior to late 1944, no thought had been given to the actual detailed analysis and construction of a sounding rocket. It was Malina's European tour in 1944 which spurred preparations for the WAC
Corporal. Malina and Stewart's 1945 study on the " 100, 000 ft. Altitude Rocket " neatly filled the gap between the Private A and the much larger Corporal. Essentially, what the WAC Corporal team did was refer back to earlier, 1938 studies
by Malina, " Amo " Smith, and Tsien for the equations and data related to the WAC Corporal 14. Malina and Tsien's formulations, in particular, were quite sophisticated. The Malina /Tsien 1943 collaboration had been a new platform built upon the earlier studies and was the fruit of more
that six years' experience in sketching the theory of rocket performance. To some extent, Malina and Stewart's 1945 work relied on past achievements : even Goddard's 1919 monograph, " A Method of Reaching Extreme Altitudes ", is listed as
reference at the back of the JPL/ORDCIT Report 15.
There is no question that their previous theoretical investigations gave them the confidence to proceed with the construction of a rocket whose dimensions and full design they had not yet completed. Most of the trajectory equations for
the Private A were differential equations, with rate changes in velocity measured as a factor of dtt. The GALCIT team had not worked through the exact formulations before, and there were several variables to account for, although the
equations were relatively straightforward 16. Tsien and Malina
made a good team. Given a specific set of launch conditions, their equations could, in Malina's own words, " completely determine the performance of the projectile. " 17 But, would these equations, calculated for a smaller rocket, accurately reflect the
performance of the WAC Corporal ? Years of experimentation, supplemented by independent theoretical study, classroom teaching, and writing on the details of 'jet propulsion' provided sufficient grounding for equations which would
approximate the WAC Corporal's flight dynamics. Malina's comment was a reasonably accurate one. 1945 was not 1938. There was a closer fit between the equations and actual performance in 1945, although some functions, such as thrust
variations in flight, were still poorly understood. Theory had evolved to the extent that mathematics could now define specific parameters of rocket performance - trajectory angles and coordinates at the summit of the
flight - more precisely. The problems lay in gathering adequate data for the complex equations the team was employing. Without radio telemetry to report back a missile's exact coordinates and functions from instant to instant, there were
large gaps in the information available for technical analysis. Such radio communications with an American missile did not come into use until the Corporal was tested in the late 1940s . 18 Much of the groundwork for the successful completion of the WAC Corporal rested upon the
mating of successful, proven rocket motors to a new casing and tail fin design. The closer fit between theory and performance came because theory evolved through a process of insight and feedback from actual experimental results.
Experimental and theoretical work in the late 1930s were simply inadequate to fly a rocket as sophisticated as the WAC Corporal. Since the WAC Corporal was an engineering system, and not a simple solid-pack rocket motor
with a steel casing, constructing it necessitated a systems approach. The WAC Corporal design and assembly team was large, with 37 members, and component work was delegated to various engineers, radar experts, and technicians. The
success of the WAC Corporal program depended upon delegation of tasks to specialist engineers. Mark Mills, working alongside an Army Ordnance engineer, Captain E. W. Bradshaw, Jr., developed the booster rocket that would
accelerate the WAC Corporal to a threshold velocity. Homer Stewart and Wei-zhang Chien calculated the projected trajectory of the missile and its ballistics (its speed and stability at various moments in its flight.) The army supplied
officers and enlisted men to develop and install radar tracking of the missile. The entire project was under the command of an Army Ordnance officer, Colonel Benjamin S. Mesick. Logistics, transport, radar, meteorology, and photographic
recording were all within the army's purview. Malina was the only civilian in a position of high administrative authority, and even he was subordinate to Colonel Mesick. As Tsien was in Europe with von Karman's Scientific
Advisory Group from April to June 20, 1945, touring German V-2 factories and interviewing engineers who had designed and built the ME-262 jet, he was not directly involved with the WAC Corporal project. However, the strategic placement of
scientists such as Mills, Stewart, and Chien in key roles meant that civilian mathematicians and aeronautics experts would actually design the WAC Corporal, finessing their theoretical calculations with the actual reality of the
constructed missile 19. The army relied on JPL engineers to produce data
on the estimated dimensions and performance of the WAC Corporal that would justify military involvement and expense. Chien, Stewart, and Mills satisfied the army's demands. The estimated overall length of the WAC Corporal
was 168 inches. The actual rocket measured 194 inches. Stewart and Chien rated the liquid propellant motor's thrust at 1, 500 lbs., and this was the delivered thrust 20. The estimated gross weight of the rocket was 7000 lbs., its actual weight, 665 lbs. However, the
engineering team estimated the WAC Corporal would carry 330 lbs. of fuel and oxidizer. In reality, the missile hoisted 400 lbs. of combustibles. This meant that the missile would have to be lighter than projected, or use more initial
thrust to attain the velocity, calculated to be 400 fps., which would allow it to fly straight up. After all, the WAC Corporal was a sounding rocket : it had to reach a maximum vertical attitude in order to accomplish its mission.
Wei-zhang Chien calculated the performance characteristics of the rocket, based upon " approximate integration of the equations of motion on the assumption that the trajectory was purely vertical " 21. The challenge was to construct a missile that would conform to these specifications.
Although the WAC Corporal did weigh less that its theoretical maximum, it carried 60 lbs. more fuel/oxidizer that its designers had projected. The trajectory of the rocket had to be vertical, or it would not reach altitude. But in order to
create a flight path " purely vertical ", as Malina and Chien had assumed, the WAC Corporal would have to jump off the " starting block " at over 272 mph. (400 ft./second). And it would have to reach this velocity in just over 0. 25 second.
So the engineering hurdles were formidable. Once again, the GALCIT rocket engineers were in uncharted territory. And once more, the team had to use engineering savvy, mathematical approximation, and the pluck or
self-assurance that their calculations were accurate in order to build a working rocket. Weight considerations were paramount. Limiting the rocket's weight might spell success for the WAC Corporal. Excessive weight or inadequate thrust
spelled disaster. It was decided that the rocket would use a modified 1, 500 lb. thrust liquid propellant motor already designed by Aerojet, the regeneratively-cooled JATO 38 ALDW-1500 22. Malina and Stewart had determined that only a liquid propellant motor, using nitric acid-aniline
combination fuel, could provide the thrust necessary to power the WAC Corporal, without adding too much weight. The team evaluated solid propellants, including ballistite (the high energy propellant used in NDRC rockets
built by Caltech during the war) and GALCIT 61-C, Parsons' improved composite propellant. Ballistite would not suffice, however, because it produced acceleration and g-forces that were initially too great, thus making the installation of
sensitive meteorological instruments impossible. GALCIT-61 would not do, either. It would produce an impulse of 77 lb.-secs./sec., but for the WAC to reach altitudes beyond 100, 000 feet, an impulse of at least 95 lb.-secs./sec. was
necessary, and this meant a motor that weighed too much 23. Sounding
balloons had already reached 100, 00 ft., and there would be little point in constructing a high-altitude sounding rocket if it could not exceed that height. The nitric acid-aniline combination suited the WAC Corporal
well. As it was hyperbolic, the propellant required no ignition assembly. The WAC Corporal engineers even added a new ingredient to the acid-aniline : furfuryl alcohol. Furfuryl alcohol, C4 H3OCH2OH, has a
slightly higher enthalpy (D Hv 107.5) than aniline (D Hv 103.7) and vaporizes at a lower temperature 24. So the fuel's energy output would be slightly greater, and its volatility somewhat higher. But even this higher energy fuel, combined with a proven liquid propellant motor, would not
suffice to lift the WAC Corporal quickly off the launch pad. The problem was a very practical one. There could be no internal guidance system aboard the WAC, because any gyroscopically controlled guidance unit would add
immensely to the rocket's weight. So the missile had to be externally guided. The launching tower had to be just high enough to shoot the WAC Corporal out a straight and true trajectory ; the instant the missile left its launcher, both the
earth's eastward motion, friction, and gravitation would begin to pull the rocket into curved flight. These forces, although physically independent of each other, would combine to lower the WAC's maximum altitude. Homer
Joe Stewart and Mark Mills devised two ingenious solutions. First, Stewart reasoned that he could reduce the rocket's weight even more by reducing the number of tail fins from four to three. The army balked at this idea. All bombs had four
fins. Three fins might not provide enough in-flight stability. But Stewart adroitly emphasized that arrows had three fins and flew quite well 25
. Mills' task was harder, and his solution even more unique. Mills knew that he had to devise a way to get the WAC Corporal off the ground fast. The liquid propellant
motor alone would not accomplish this ; the rocket, under power of the 38 ALDW-1500 motor alone, would rise slowly. Even with a high launch tower, it would soon veer away from the vertical, as many of Goddard's rockets had done in the late
1930s. The solution was a booster rocket. Clusters of NDRC rockets, attached to the Private A, had launched that rocket quickly and dropped off at the end of the launch ramp. The same type of unit could be mated to the WAC Corporal. But
there was one problem : the WAC Corporal was meant to be a vertically launched rocket ; the private A launch ramp had been inclined off the horizontal. The WAC Corporal booster rockets had to be designed so that the rocket itself would
leave both the launch tower and its booster rockets behind. Since the WAC Corporal would stand upright under its launch tower, Mills, Bradshaw, and the " Booster Group " faced a dilemma. If they placed the booster rockets
on top of the WAC Corporal, they would have to install explosive bolts to remove the booster rockets below the WAC Corporal to remove the booster assembly before the missile left its launch track. The blast from the booster rockets might
very well sear through the WAC Corporal's casing and ignite the fuel. To install the booster rockets below the WAC Corporal meant designing an escape mechanism, using bolts that would align both the booster and the WAC piggy-back fashion.
Mills planned his design so that the booster would drop off at the top of the tower. This was possible, of course. Mills and his crew designed a set of rods with a circular ring at the top, placing the entire apparatus at
the bottom of the WAC Corporal (below its exhaust nozzle) ; they then secured the booster rocket assembly to this ring. The ring fitted snugly below the rim of the WAC Corporal's fuselage. But the problem was not so quickly resolved. The
WAC and the booster rockets would be firing simultaneously. The WAC's exhaust just might burn through the booster rockets' casing and ignite them, if there were no heat ablative shield above them. So the Booster Group
designed one. The booster rocket, dubbed " Tiny Tim ", was short, black, and had three fins, aligned with those of the WAC Corporal just above it. Clustered in its tail were up to 24 modified NDRC ballistite rockets. Technicians could
regulate the burning time of " Tiny Tim " by plugging the exhaust nozzles of some of the NDRC rockets. If the atmospheric pressure and temperature on a particular launch day were too low, selected booster rockets could be plugged, thereby
reducing the overall thrust 26. Mills wanted to ensure that the WAC
Corporal would not leave its tower too fast. Mills and Bradshaw had mated two rockets together. They had created an early version of the " step rocket ". Now, the WAC Corporal team had the outline of a sounding rocket,
one designed quite ingeniously. In mid-1945, the WAC Corporal rested at the top of an engineering pyramid, the base of which the GALCIT team had an established network of technicians and engineers. Given a ready source of research funds,
JPL engineers were able to convince Army Ordnance that their ideas would work. Theory was encapsulated in the process of developing and writing " Letter Order Contracts ". These short contracts were essentially research proposals, and
military officers served as the review board evaluating them. The army expected sound theoretical work which would establish clear performance guidelines. But these " Letter Order Contracts ", of necessity, had to include
some experimental data. As Malina wrote in " Consideration of the Feasibility of Developing a 100, 000 ft. Altitude Rocket " : "The calculations of the performance of the rocket are based on earlier
analyses of the sounding rocket carried out by various investigators. The parameters used in the present calculations are based on present day experience with propellants and rocket unit components and recent data on the
drag coefficient of projectiles 27".
Such " earlier analyses " had been purely theoretical and included Robert Goddard's " A Method of Reaching Extreme Altitudes ", Willy Ley's " Les Fusées volantes météorologiques ", as well as Malina and 'Amo' Smith's own 1938 work,
" Flight Analysis of the Sounding Rocket ", and Tsien and Malina's study on propulsion by " successive impulses " 28 . Malina and Stewart made sure that the literature consulted, while not voluminous, reflected well-established work and their own prior research. True to form, Malina's
" Consideration of the Feasibility of Developing a 100, 00 ft. Altitude Rocket " appeared at first to be largely theoretical. Although the choice of propellants had been made on the basis of experimental evidence, in January 1945,
when Malina's memorandum was completed, Malina and Stewart had not yet built a single WAC Corporal. The project's main goal shaped the missile's specifications : construction of a relatively small rocket with a narrowly-defined mission -
exploration of the fringes of space. The point was to calculate the trajectory of a missile expected to reach " extreme altitudes ", as Goddard so tersely put it in 1919. With a motor chosen from the working inventory of Malina's own
company, Aerojet, a motor with an known thrust rating, Homer Joe Stewart concerned himself with launch and flight velocities. Stewart went to work, calculating the stability of a missile in " vertical flight ". He and
Malina believed that, by discovering the minimum deviation from the vertical in flight, they could calculate the optimal initial lift-off velocity of the rocket. This initial velocity, Uo, could not be too high, for, as previously noted,
too severe a take off speed would spell disaster for any scientific instruments on board. To determine the optimal value of Uo, Stewart calculated a series of ratios, q / qo where q represented the angle deviation from the vertical during
flight and qo = the rocket's angle of deviation from the vertical just prior to launch. qo = 1 when the rocket stood on the ground, for, as Stewart and Malina wrote, " it is probably not practical to expect the deviation angle at
launching to be less than 1°. " For any simple multiple of Uo - 100, 200, 300 ft./sec., there had to be a ratio, q / qo, which theoretically expressed the degree of divergence from the vertical the rocket might assume in flight.
Stewart's use of this ratio of trajectory angles, q / qo, meant that two key variables would determine optimum initial launch speed : velocity in flight and the angle of the rocket's path upward. The
smaller the ratio, the more vertical the flight path. Stewart wrote out an equation which appeared to be simple and straightforward :
where U= the flight velocity g= gravitational force, and t = time
In other words, a change in the rocket's trajectory equaled its velocity - increasing steadily as gravitational force decreased - multiplied by its angle of deviation from the vertical.
Of course, Stewart knew that, to maximize the rocket's altitude (given constant thrust and acceleration), it was important to maintain the trajectory as close to the vertical as possible. In other words, the rocket
had to fly nearly straight up during its powered phase, to reach as great height as possible. The objective was not to have the WAC Corporal fly in an arching trajectory like an artillery shell, but for its parabolic path to
peak above 200, 000 feet. (This, of course, meant that the rocket would land fairly close to its launch point.) Now, velocity increases linearly with time, so U=Uo
+at (a = constant acceleration) This is the case because 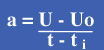 if, ti = 0 and t = any arbitrary later time . 30
(Here, Uo = some arbitrary initial velocity.) Therefore, 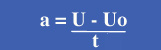 and consequently, at = U - Uo. Finally U = Uo + at
Taking the derivative of q as a function, not of time, but of velocity, Stewart resolved the equation
into ____
Ud q / d U - [g/a] q = 0 He could do this because d q / d
U is a function of both trajectory angle and velocity. But of these will determine the rocket's maximum altitude. Velocity equals dx/dt, (v = dx/dt)
with x = a vector in any direction. Stewart knew that a rocket's trajectory in the x direction was a vector
equal to 90° minus its deviation from the vertical (90° - q ), and as, v = v0 cos (90 - q ), and v = U
therefore, U = U0 cos (90 - q ) or U = U0 sin q and U = dx /dt
31 This equation, which represents the merging of two different forms of
vector analysis, indicates that the rocket's velocity would be a function both of time and the angle of deviation from the vertical during flight.
Now, from the original equation , as gravitational force diminished every instant the rocket was accelerating upward, g/U became smaller and smaller - in fact, it became a constant acceleration. ( This also implies that d q / dt was approximately equal to some
multiple of q. ) Since d q / dt, then, is a function o time, and velocity and acceleration are both functions of time, d q / dt and dU / dt must be related to each other. They are connected by the Chain Rule, and so,
( d q / dt ) x ( dt / dU ) = d q / dU 32 Then if, U = Uo = at when this equation is differentiated, dU = adt Both sides of this equation can be divided by a : dU/a = adt
The result, dU / a = dt, of course, can be substituted into the equation
to give ___
Which simplifies to  The entire equation then becomes : 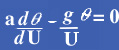 Multiplying this equation by (U/a) gives 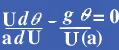 and by cancellation , 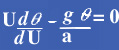 In this equation, Stewart assumed that velocity was a constant : (U = a ).
Stewart achieved the final steps by re-iterating that flight trajectory was fundamentally a function of velocity, the force of gravity (g) - which was becoming more and more negligible as the rocket ascended - and q.
By integration, q / q 0 = [U / U 0 ] g / a or q / q 0 = [ 1 = at / U0 ]
g / a The steps leading to the above results begin with the equation : 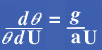 Dividing both sides of this equation by U, the next step is __ 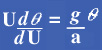 Then, dividing this quantity by q, the resolution of the consequent step is :  Lastly, before integration is performed, multiplication of both sides by d U brings:
The next step in Stewart's derivation of the above ratio, q / q 0 , involved indefinite integration, using the natural logarithm, ln : ln q ¥ = ln U (g/a) Which unfolds to ln q = ln U ( g / a )
However, this is none other than a form of the natural exponential function, e ln q = e ln U (g /a)
Because, as a general rule, e xy = (e x ) y 33
Therefore e ln q = (e ln U )(g /a)
(here y = g/a and x = ln U) and because (e ln U ) = U (any e ln x = x)
thus (e ln U) ( g/a ) = U ( g/a ) But since e ln q = (e ln U) (g/a)
(see above) e ln q = q, it is clear that U (g / a ) = q
What followed for Stewart was the creation of the ratio [ U / U 0 ] (g / a ) = q / q0 (g /a) And since from the equation for velocity ,
and, 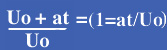 The final result for Stewart was : q / q
0 = [1 + at / U0 ] g/a 34
Returning to the equation, Stewart reasoned that if g / U constantly decreased as the rocket gained in altitude, then the deviation of flight path from the vertical d q / dt
would also decrease - that is, the flight path would not diverge too much from the vertical, as gravitational force weakened. Of course, there would always be some deviation, the result of air resistance, wind
gusts, vibration, etc. So, Stewart had to find a value for q / q 0 that was as close to the ideal as possible. Now, the degree of divergence from the vertical at the apogee of the
rocket's flight was actually proportional to the initial lift-off velocity, for as Stewart calculated q / q 0 = [1 + at / U0 ] g/a
setting the exponent g/a = 1, then q / q 0 = [1 + at / U0 ]
Since the " height obtained during coasting [ at the end of powered flight ] is proportional to cos 2 q {cos 2 q = [cos q] 2}, where q is the
angle from the vertical at the end of burning ", if q = 20°, then (cos 20°) 2 = 0. 883, equivalent to a 12% loss in height 35
. Because Stewart and Malina " wanted to limit the inclination at the end of burning to about 15°, " - about a 10% loss in maximum altitude - they had to find
corresponding initial velocity, U0, which would conform to this expected figure . 36 For a set of initial velocities, U 0, with g /a = 1, Stewart derived the following data :
These data show that the higher the initial velocity, the less the
deviation from the vertical in flight. Since an initial velocity of 500 - 600 ft/ sec. would be too high, i.e. would create g-forces beyond the stress limits of the meteorological payload, Stewart chose the value q / q 0 =
15.2 as the outside limit for trajectory deviation. This would have meant an initial liftoff velocity of approximately 100 fps., too low a figure .
37 The actual initial liftoff speed of the W AC Corporal was close to 400 fps., with q = 4.5. 38
There was no way to guarantee that a meteorological accident, such as a sudden wind burst at altitude, or mechanical vibration, would not alter the missile's pitch in flight. Stewart had to assume ideal conditions for
his calculations to have any validity. He had to design the WAC Corporal for stability and low weight. These two variables were at odds, for certainly, the heavier the rocket, the less it would be buffeted by winds
during flight. Added weight did not necessarily correlate with increased stability, as the Germans had discovered in the A-4 tests. Design and the behavior of the rocket as it approached and passed
transonic speeds would also have determined the failure or success of a WAC Corporal flight. Given advantageous meteorological conditions in the New Mexico
desert - generally dry and cloudless - and Stewart's economical design of the WAC Corporal fuselage and tail assembly - his calculations were remarkably accurate. His values for the initial velocity, Uo, were
arbitrarily chosen, in simple whole number multiples of 100 ft/sec., and he assumed no deviation from the vertical in flight ; in point of fact, the WAC Corporal flew straight and true. Of course, the story was a bit more
complex. Lacking an internal guidance system, the WAC Corporal team had to construct a launch tower approximately 100 feet high to ensure that the rocket would reach launch velocity without toppling over soon after it flew in open air.
Stewart's calculations provided more than theoretical reassurance that the rocket might fly correctly. His determination of launch velocity and optimal deviation from the vertical in a wind-less sky gave Malina and
the WAC Corporal team guidelines to help determine the amount of fuel to be carried, the tower height, and a reasonable calculation of the altitude the rocket would reach. Not inconsequentially, the
mathematical data also convinced Army Ordnance that the WAC Corporal was worth funding. " Considerations of the Feasibility of Developing a 100, 000 Ft. Altitude
Rocket ", short as it was (12 pages, excluding charts and diagrams), represented a landmark in the evolution of Frank Malina's (and Homer Joe Stewart's ) thinking. Malina truly had left behind von Karmans's
mantra : theoretical, mathematical proof first before experiment (although he would still have been loathe to admit it). The paper is heavily theoretical, but laced through it, Malina and Stewart gracefully
admit their dependence upon prior experimentation as the basis for key decisions about the WAC's design and propellants. Just after Stewart's calculation of optimal initial velocity ends, Malina added the following qualification :
" For a constant thrust rocket unit, the simplest kind to construct, the net acceleration is not constant, but increases as the propellant is consumed, so the results of the preceding calculation... are not strictly
applicable to this case, but the results can be applied by using a mean acceleration in which the initial acceleration is weighted more heavily than that during the later part of the burning period. " 39 The dynamics of rocket flight were too complicated for the first-order
differential equations Stewart had devised. The WAC's acceleration was not constant, but varied throughout the flight. If Malina and Stewart had not approximated the projected velocity, they would have been left
writing data that did not conform to true flight specifications. Actual flight performance might differ markedly form their predictions. From instant to instant, the WAC Corporal would have been increasing its
velocity as it became lighter and lighter. Therefore, only higher order equations would have approximated the true state of the rocket's motion from second to second.
Malina and Stewart could have used higher order derivatives, such as second derivatives, to find the mean acceleration of the WAC Corporal. Yet they did not, leaving such calculations to other engineers in the
future. They gave no explanations as to why they were more precise and detailed in their calculations ; undoubtedly, they were pressed for time, as their report appeared in print less than one month after
Malina's return to the United States. Malina wrote in his memoir of the ORDCIT project : " The Army Ordnance Department requested that JPL study my proposal for a sounding
rocket. It was quickly completed and submitted on January 16, 1945... " 40
Malina had returned to the United States from his European tour in mid-December 1944. When Malina contacted Colonel Trichel in Washington (before traveling back to Pasadena) and told him of his new
idea, Trichel's response was immediately favorable. Malina had, in fact, sold him on the concept of a scaled-down version of the Corporal as " a first step in the development of a guided anti-aircraft missile "
41. In early January 1945, priorities for Malina and Stewart involved
convincing the army to fund their rocket. One did not need complex mathematics to do so ; one only had to prove to the army's satisfaction that the rocket could fly.
Precise technical description of the WAC Corporal's flight, however, would have involved more detailed mathematical structure in their paper. Essentially, the type of motion Malina was measuring was not
strictly linear. The rate of fuel consumption would have been changing from moment to moment, and there was no way to measure the actual conditions inside the fuel chamber. Even assuming a constant feed rate
of propellant into the chamber, Malina could not have predicted that the burn rate would remain constant as the rocket accelerated. If the burn rate did remain constant, then it would be safe to say that the WAC's
velocity would have been impossible to predict accurately, as the missile would have been accelerating every single moment until its motor cut off. Stewart and Malina had to assume a minimum initial
velocity, and predicate their trajectory calculations upon that value. To have described the WAC Corporal ballistics as precisely as possible would have necessitated a computer. In 1945 America, computers were
not enlisted in the effort to calculate rocket guidance. The Mark I, a digital computer developed at Harvard for the navy and the NDRC, was operating as early as 1944, carrying out complicated differential
(ballistics) calculations. The Office of Naval Research, created in 1946 by the War Department, soon began funding digital computer research at MIT, the Raytheon Corporation and other venues. MIT's Whirlwind (on
line by 1951) and Raytheon's Hurricane were the fruits of this work. But engineers had no access to such machines. In fact, Homer Stewart used an adding machine to help him with his trajectory calculations. Nothing
more sophisticated was available to him . 42
Computer guidance of missiles during wartime had made strides under the Nazis. Helmut Hoelzer, a civilian enlistee who worked at Peenmünde after October 1939, developed a prototype analog computer used to
test guidance systems' accuracy before they were installed in A-4 missiles. Hoelzer wrote a two-part dissertation on his analog computer in 1945 - he divided the thesis into two parts because US Army
occupation authorities would not let him publish a work on computer application to rocketry, very much considered a military application in post-war Germany. Hoelzer then re-submitted the thesis, with all
references to rockets in the second part only. He showed only the latter half to his professors at the University of Darmstadt. After receiving his doctorate in 1946, Hoelzer emigrated to the United
States, where he went to work under Wernher von Braun at the army's Redstone Arsenal in Huntsville, Alabama. In the early 1950s, an American electronic expert who had worked with Hoelzer at Hunstville,
James Farrior, developed an analog computer which could correct for sideways drift and range control problems on the Redstone rocket. This machine was the first computing device installed on any American missile 43. Further research on digital computers in the United States
would have to await work done at the Defense Advances Research Projects Agency (DARPA), founded in 1958, and at NASA/ Both of these organizations went into overdrive in their research work, following the launching of Sputnik in 1957
44
In 1945, what saved Malina and Stewart from the necessity of hair-splitting calculations was one simple fact : to attain a minimum altitude, the engineer need only know a minimum threshold velocity.
The longer a rocket is in flight, the greater the possibility that some anomaly, either mechanical or dynamic, will push it to diverge from a predicted flight path or anticipated performance. There are too many
variables at work simultaneously for the projectile to function exactly as predicted. Burn rates can fluctuate ; the rocket can roll on its vertical axis, thus changing its path as it turns ; pumps can vary the flow rate of
fuel to the chamber as the rocket lightens ; the very chemistry of combustion can change during flight. Rocket engineers in 1945 could only predict the gross behavior of the machines they were constructing.
Certainly, Malina would not have confidently predicted the theoretical success of the WAC Corporal, had he not felt reasonably sure that his and Stewart's mathematics were on the mark. Notably, the WAC
Corporal flew on a nearly vertical path for every one of its ten test rounds. However, the powered segment of its flight was short, on the order of 45 seconds. Nevertheless, in 45 seconds a great deal can
happen inside a rocket motor. There was no guarantee that any flight of the WAC Corporal would succeed, even though a proven rocket motor had been installed in the missile. The WAC flew well, not merely because
it had no defined target, but because Malina's crew had systematically constructed a machine that would perform within estimated performance parameters. The rocket motor's design had been tested
over a period of more than two years. The WAC's engineers had control over several flight variables : overall weight, type and amount of propellant used, design of the motor, pumps, and aerodynamics. Even
so, each new flight was an adventure. However, the reliability of the WAC power plant and auxiliary systems was not a matter of luck. These systems, mechanically speaking, would
have proven quite durable, even if the WAC Corporal had flown for several minutes. It was still possible for some type of anomaly to develop within the fuel combustion process, but at what exact instant
chaotic fluid behavior might have set in depended upon the threshold for instability within each particular motor. The WAC Corporal began on paper in mid-January 1945. Eight months
later, almost to the week, the first fully operational WAC flew at the White Sands Proving Ground. From vision to reality in less than a year - the pattern of quick construction, with theoretical support sometimes
tagging along - this had been a hallmark of GALCIT work ever since the late 1930s. But there was a hint of an added incentive to rapid testing of the WAC Corporal, for Malina knew of the coincidence between the
detonation of atomic bombs over Japan in August 1945 and his own missile work 45. Clayton Koppes, in JPL and the American Space Program, noted that the " [army] ranked guided missiles second only to nuclear weapons as a military priority " at the end of the war. " 46
October 11, 1945 was a momentous day for Frank Malina and the entire crew. Four rounds had been fired previously, but they had been " dummy rounds ", the nose cone filled with concrete, or the missile
only partially filled with propellant, to check the reliability of the motor. On that day, a Thursday, from a control house 465 feet away from the launch tower, a member of the " Missile Firing Group " of the WAC test
program sent an electrical signal to the base of the WAC (through a circuit attached to the ballistite rockets), and the missile was on its way 47
. " We craned our necks to watch the WAC's smoke trail until the engine stopped at around 80, 000 ft ", Malina wrote exultantly.
" On the basis of radar tracking data, it was estimated that the maximum altitude reached was between 230, 000 and 240, 000 ft. The total time of flight was about 450 seconds or 7.5 minutes. The velocity
of the WAC at the end of burning was about 3, 500 feet from the launcher, which meant that the WAC had maintained a very satisfactory vertical path. Success! "
48 Malina had launched his sounding rocket. Despite his intensely
controlled demeanor, he could not hide his joy. Even his retrained, matter-of-fact description revealed a wealth of information. The first implication of his short triumphant note was that data on the flight was
quite imprecise. The zenith was " between 230, 000 and 240, 000 feet... Velocity was about 3100 ft./sec... ; the impact was about 3, 5000 feet from the launcher. " Translation : there were limits on the accuracy of
data the WAC crew could collect on the flight. Some of the numbers are startling. If the WAC Corporal peaked out a over 230, 000 ft, it reached an altitude of over 43. 5 miles. It was nearly
in outer space, as the threshold with space lies at approximately 50 miles above the earth's surface. At 3100 ft./sec, the rocket was traveling at 2113. 63 mph., or Mach 2.8, nearly three times the speed of
sound. What kind of buffeting had the rocket endured as it passed through the sound barrier ? There was no way for Malina to tell. The WAC's shell, minus its nose cone, landed 3, 500 feet from the launch tower- two-thirds of a mile away.
The nose cone, of course, presented a dilemma. The engineers decided to equip the WAC with a parachute, so that its meteorological instrumentation could be salvaged. As the WAC passed through its flight
apogee, a small gyroscope in the nose cone detected deviation from the vertical and closed an electrical circuit to a set of explosive bolts. The cone then was supposed to separate from the missile's fuselage. There
was also a mechanical clock in the payload bay, as well as a radio link to the Army Signal Corps Group on the WAC team ; theoretically at least, there were three systems for retrieving the nose cone.
Six fully operational WAC Corporal flights lifted off in 1945, and not a single one of them properly deployed the nose cone parachute. No matter, reasoned Stewart. The nose cone wouldn't land near the ground
crew, anyway. He was sure the " probability of being struck was extremely small. 49" So, many of the crew just milled about, hoping to see the nose cone float down at a relatively leisurely pace. Instead, on one shot, the cone came crashing in at over 2000 mph., two hundred
yards from where the men were standing 50
. The parachute had failed to deploy, and true to form, the WAC Corporal had landed close to its lift-off point. Ten years of bizarre mishaps had inured the GALCIT rocketeers to a " touch of drama ".
Statistically speaking, the WAC Corporal shots were completely successful. Out of six operational tests, there was not a single failure. The tenth lift-off was the most dramatic, as it was a night flight,
Thursday, October 25, 1945. But, again, the parachute refused to work properly. " This did not surprise us ", Malina blithely commented. " There was very little experience on the behavior of chutes at high altitudes. " 51
Malina and the WAC team soon made plans to remedy this deficiency. Several recommendations to Army Ordnance on redesign shapes a new agenda: construction and test firing another round of WAC Corporals
later in 1945 or early in 1946 ; included on the list was a call to redesign a " reliable nose cone release mechanism and... a stronger attachment of the parachute to the vehicle. " 52 Following a strategy session among JPL engineers, Army Ordnance, and Army Signal Corps personnel
in Aberdeen, Maryland, November 9, 1945, a new round of WAC Corporal tests was scheduled for the next year. The White Sands Proving Grounds was the scene of these tests in December 1946 and February-March 1947.
The WAC Corporal had proved its mettle. The next generation sounding rocket, the Aerobee, designed by Aerojet and assembled at Douglas Aircraft's Santa Monica, California plant (where the WAC had seen the
light of day) was its direct descendant. The Aerobee broke ranks with the WAC Corporal in one significant respect : it became a vehicle devoted solely to the scientific exploration of the atmosphere, and was
not the progenitor of a new class of military rockets. The Aerobee, first launched on November 24, 1947, was a Navy Bureau of Ordnance-funded project. The director of the Aerobee program, however, was James A. Van Allen,
of the High Altitude Research Group of the Applied Physics Laboratory at Johns Hopkins University. Van Allen had visited Aerojet in 1946, " to survey its rocket capabilities. " When he returned East, he enlisted navy
support for the construction of the Aerobee; A 45AL-2600 Aerojet liquid propellant motor powered Aerobee. This motor used the same RFNA-aniline-furfuryl alcohol fuel that had filled the WAC Corporal's
tanks. The Aerobee even had the same type of booster rocket (the " Tiny Tim " variety, but lighter, with a higher thrust), mounted below the Aerobee's rocket motor, as was characteristic of the WAC 53 The WAC-Aerobee connection generated a secondary benefit : for the
first time, West Coast rocket technology was migrating to the East, rather than the other way around. The effort to build a rocket for peaceful scientific uses had become nationwide. Aerobee would hoist
instrumentation into the upper atmosphere well into the 1950s. Malina could feel justifiably proud. His work had spurred the development of a rocket whose design was not meant to be mated to an explosive.
When Malina watched the first fully-charged WAC Corporal soar into the sky at White Sands on October 11, 1945, he may have felt himself present at the birth of a new area in rocketry. " In the astronautical
fraternity ", he wrote in 1971, thinking back on that cloudless day in the New Mexico desert, " the tradition of lighting up a cigar had not yet begun. We contented ourselves by letting out whoops of joy, shaking
hands, and patting each other on the back. Our celebration was of short duration. We quickly returned to work to prepare for the launching of the next round of the WAC the next day! " 54 Malina's use of the term " astronautical ", rather than " rocket " is
noteworthy. There is a distinct difference between rocketry and astronautics. Astronautics concerns itself with exploration of extraterrestrial space. Rocketry is the science of design and
construction of vehicles with a self-contained power and oxygen source, operating on the reaction principle. In 1971, when Malina wrote these comments, astronautics was a well-entrenched field of science and
engineering. But it is a vastly broader field than rocketry. Astronautics as a scientific endeavor had not come to fruition in 1945. Malina's " slip-of-the-pen " reveals, at the least, an expanded vision of the fruits
of his work. In truth, Malina, and the WAC Corporal team had turned a page in the history of engineering. Ironically, none of the other original members of the GALCIT Rocket
Team was present when the WAC Corporal lifted off in 1945. John Parsons, the co-inventor of the RFNA-aniline fuel, along with Malina, had left Aerojet that very year to return to independent work in the
explosives industry. Parsons died tragically only seven years later, in 1952, at age 38. Hsueh-shen Tsien was working with von Karman in Washington, and preparing to move to MIT ; not even Martin
Summerfield, who had worked on ORDCIT Project, was at White Sands that first September day. Four years later, in 1949, he would leave JPL for Princeton University, where he became a professor of Rocket Propulsion Technology.
Of the original GALCIT team, Malina alone stood and watched the successful tests of the WAC Corporal in 1945. His presence may be taken as a symbol, not only of the continuity of hard, determined
canyon known as the Arroyo Seco, but also of the Rocket Team's metamorphosis. As in 1937, the GALCIT Rocket Team drifted apart ; but this time it was forever.
The WAC Corporal tests represent the maturation of American rocketry in the waning years of World War II. From an engineering standpoint, the WAC remains a remarkable achievement. Although it rested
squarely on the effort of university-trained engineers, a research team organized by private industry and the financial and organizational contributions of the US military, developing the WAC was an effort
without any input from an international community of scientists. Like the V-2, the WAC Corporal was the product of a state-funded project, one which fused the collaborative work of industry, the army,
and academia, Malina's " WAC team " conducted all its research and directed construction completely in secret. As there was no chance of input from German engineers, the creation of the WAC Corporal was
strictly an independent accomplishment. Malina's crew had come to its design decisions without the benefit of the only other intensive rocket-building project in the world : that of Nazi Germany. Malina never
got a complete look at German technology during his trip abroad in 1944. He compared notes with British rocketeers, but they, too, were leery of divulging too much. Not even the amateur rocket societies in
the United States were involved. So, the WAC Corporal was born entirely of Caltech and army know-how. Perhaps, if Tsien had been a member of the WAC Team, theoretical
calculations describing the WAC's behavior in flight would have been even more detailed and accurate than they were. Nevertheless, Homer Joe Stewart's contributions gave Malina all the underpinning he needed
to convince himself and the army that the WAC could fly. GALCIT and Aerojet engineers had confronted and mastered many theoretical and design problems in building their early rocket motors. Others remained
to be solved (the problem of motor throbbing due to unstable burning, and the use of newer more sophisticated, more refractory materials in rocket motor casings, such as ceramics and titanium.)
Unquestionably, the calculation of the WAC's performance could have been more precise. Because tracking capabilities at White Sands were rudimentary in October 1945, it was impossible to do more than
estimate results. Had the WAC Corporal's motor been in powered flight for more than a few minutes, other mechanical and dynamic problems might well have surfaces. Yet, the WAC Corporal used proven
technology, and within the limits of its mission, it performed quite well. In the WAC Corporal, GALCIT had created the first of a new generation of rockets. Its design mated theory to construction. By 1945, both GALCIT
and American rocket technology had entered a new and challenging phase.
1 . Malina (1971), p. 361.2 . ORDCIT Memorandum No. 2 (Attributed by Malina to H.S. Tsien) " Research
Program for the Second Type of Long-Range Jet Propelled Missile (XF30L 20, 000) ". Jet Propulsion Laboratory/GALCIT, California Institute of Technology. August 20, 1944. JPL Archives, Document No. JPLH5, 3-142. The memorandum
bears the signature of Theodore von Karman, but the GALCIT Director merely signed off on the report as a formality. The Army Ordnance Contract sponsoring this " feasibility study " was contract No. 04-200-ORD-455-RAD, 2865-1. 3 . Koppes (1982), p. 39.
4 . ORDCIT Memorandum No. 2 (1944), pp. 1-2. 5 . Von Karman (1943), p. 3. Phase 3 called for the " design and construction
of a projectile of 10, 000 lbs. weight or larger with a range of the order of 75 miles. " 6 . Frank J. Malina and Homer J. Stewart. " Considerations of the Feasibility of
Developing a 100, 000 Ft. Altitude Rocket (The " WAC Corporal "). ORDCIT Project, Letter Order Contract No. W-04-200-ORD-455, ASF Ordnance Department, Memorandum No. 4-4, JPL/ GALCIT, January 16, 1945. JPL
Archives, Document # 3-121, Microfilm # 614-232, Item 33. 7 . Malina (1971), pp. 359, 364. 8. Malina (1971), p. 364. 9 . Malina and Stewart (1945), p. 1. Malina wrote that WAC Corporal " would serve as a scaled-down model of the " CORPORAL ", which is now under development, and for this reason,
the designation " WAC CORPORAL " has been chosen. " 10 . Malina and Stewart (1945), p. 1. 11 . Malina (1971), p. 358. 12 . Malina and Tsien (1943), pp. 15-17. For the equations relevant to Corporal performance, see pp.
20-25 of the memorandum. 13 . Malina and Tsien (1943), p. 13. 14 . These monographs included the work by Malina and Smith, " Flight Analysis of the Sounding
Rocket " (1938), and Tsien and Malina's " Flight Analaysis of the Sounding Rocket with Special Reference to Propulsion by Successive Impulses " (1938). 15. Malina and Stewart (1945), p. 12. 16 . For a more detailed perusal of the equations, see Malina and Tsien (1943), particularly p. 16. Most of these equations were non-lienar, that is, they did not take the form AX+By+C=0. W. Z
Chien's 1944 on the Private A " Rocket Missile XF10S1000 "), JPL Document #614-232, Item 34, also contain several of these trajectory equations. 17 . Malina and Tsien (1943), p. 16. 18 . Koppes (1982), p. 40.
19 . Malina (1971), p. 366. 20 . It should be noted, however, that the estimated and the rated thrust of the WAC Corporal
matched so neatly because the engineers were using an already proven motor in the missile, the 38 ALDW-1500, built by Aerojet. 21 . Malina and Stewart (1954), p. 8. 22 . See : Aerojet Marketing Brochure (1948) " Experience Aerojet : The Accomplishment ", p. 50.
The 38 ALDW-1500 was originally designed as a JATO unit for the Navy Bureau of Aeronautics, and was meant to be used on flying boats. The motor delivered its rated thrust for 38 seconds, and
Aerojet delivered 100 of the units to the navy by May 1945. 23 . Malina (1971), p. 359. The impulse/weight ratio of lbs.-seconds/second indicates the rocket's
thrust potential by stating it in terms of the number of lbs. of thrust generated per second, for every second the motor is in operation. Thus, a GALCIT 61-C motor producing 77 lb. secs/sec. of
thrust would produce 77 lbs. of thrust per pound of propellant burned for every second in operation. In effect, thrust potential is a good measure of the rocket's acceleration, which is the change in velocity/ unit time. 24 . Lange's Handbook of Chemistry, 11th Edition. (1973). pp. 78-84-85, 10-200. For vaporization temperatures, see pp. 9-97, 9-101 25 . Malina (1971), p. 361. 26 . In static tests of the booster rockets, Mills'crew determined that the lower the atmospheric
temperature, the longer the burning time of the rockets. For example, at 110° F. , the burning time was 0.756 second. At 30° F., the burning time was (on average) 0. 800 seconds. By plugging
selected booster rockets within the cluster, burning time could be reduced, so that the " Tiny Tim " booster did not produce excessive thrust. See : Capt. E. W. Bradshaw, Jr., and Mark Mills.
" Developement and Characteristics of the WAC Corporal Booster Rocket ". Project Note No. 4-30. Jet Propulsion Laboratory, California Institute of Technology, February 26, 1948. JPL Archives, Document #PN 4-30, p. 9. 27 . Malina and Stewart (1945), p. 2. The Army Ordnance " Letter Order Contract " No. was #W-04-200-ORD-455, the same contract funding the Private A. 28 . Malina and Stewart (1945), p. 12. Goddard's work appeared originally in the
Smithsonian Miscellaneous Collections, Vol. 71, No. 2 (1919) ; Ley and H. Schaefer's " Les Fusées volantes.. " appeared in L'Aerophile, vol. 44, No. 10 (1936). p 228; Tsien and Malina's study, more technical
than Malina's work with A.M.O. Smith, was published in the Journal of Aeronautical Science, Vol. 6, No. 2 (1938). p. 50. All these works, including Smith's " Flight Analysis of the Sounding Rocket ",
Journal of Aeronautical Science, Vol. 5 (1938) have been cited above. 29 . Malina and Stewart (1945), p. 7. 30 . Young (1992), p. 39. 31 . Young ( 1992), p. 64.
32 . The Chain Rule relates two derivatives with different variables in a composite function by relating the first derivative to the second derivative through a middle variable which 'connects' the
two. In Leibniz notation, dy/dx = (dy/du) (du/dx) . See : Varburg and Purcell, Calculus, with Analytic Geometry, 6th Edition. Englewood Cliffs, NJ, 1992, pp. 123-130. 33 . The natural exponential function, e, is defined as f (x) = e
x, and represents an irrational number approached as one derives the quantity (1 + 1 / n ) n (as n increases to infinity ). Leonard Euler
(1707 -1783) first calculated e to several decimal places : 2. 71828183. See : Aufmann, Barker, and Nation. College Algebra and Trigonometry, 2nd Edition. Boston, Houghton Mifflin, 1993, p. 255. 34 . I am indebted to Mr Yibao Xu of the Dept. of History of Science, City University of New York
Graduate Center, for his gracious help in clarifying certain key points in Stewart's derivation of the optimal flight path for a sounding rocket. 35 . Malina and Stewart (1945 ), p. 7. 36 . Malina and Stewart (1945 ), p 7. 37 . For Stewart's own derivation of these equations and data, see : Malina and Stewart (1945), pp.
5-7. Stewart chose a flight time of t = 44 seconds because he knew the specifications of the Aerojet motor and could calculate that at 1500 lbs. of thrust, the rocket would require 40 50 seconds to
reach an altitude of 100, 000 ft. The deviation ratios listed above may be found on the same page. 38 . Malina and Stewart (1945), p. 8. 39 . Malina and Stewart (1945), p. 7. 40 . Malina (1971), p. 359. 41 . Malina (1971), p. 353. 42 . Paul N. Edward. " Why Build Computers ? " in,
Major Problems in the History of American Technology, Merritt Roe Smith and Gregory Clancey, eds. Boston, Houghton Mifflin, 1998, pp. 456-457. The article is excerpted from Edwards' 'The Closed World : Computers and the Politics of
Discourse in Cold War America. Cambridge, Mass. MIT Press, 1996. In an August 1998 interview with this research, Dr. Stewart claimed that, even with the help of an adding machine, the WAC Corporal calculations took days to complete. 43. Paul Cerruzzi. Beyond the Limits, Flight Enters the Computer Age. Cambridge, MIT Press, 1989, p. 86. See also : Understanding Computers : Space. Time-Life Books, eds. Alexandria, Virginia,
Time-Life Books, 1987, pp. 8-13. Information on Hoelzer's work at Peenemünde may also be found in Neufeld (1995), pp. 104-107. An analog computer uses the movement of gears or voltage
fluctuations, that is, continuous variables, to measure data. However, a digital computer records data in discrete on-off bits, thus basing its technology more fundamentally on quantum mechanical principles. 44 . Jeffrey Bingamam and Bobby Inman. 'Dual-Use Technology, 1991. ' In, Smith and Clancey,
eds., (1998), p. 446. 45 . Malina (1971), p. 364. Malina wrote, after viewing the test site of a bomb blast sometime after
September 26, " It was a very disturbing sight, especially for us who were involved in the development of long-range rocket missiles. " 46 . Koppes (1982), p. 24. 47 . Malina (1971), p. 361. For a description of the WAC Corporal booster ignition method, see :
Bradshaw and Mills (1948), p. 13. 48 . Malina (1971), p. 365. 49 . Malina (1971), p. 366. The quote is Malina's. 50 . Malina (1971), p. 366. 51 . Malina (1971), p. 366. 52 . Malina (1971), p. 367. 53 . Aerojet : The Creative Company
(1997), p. III-7 ; III-36. A photograph of an Aerobee launch appears on p. III-39. See also : Koppes (1982), p. 24. 54 . Malina (1971), pp. 365-366.
|